SEDIMENTOLOGY Lab.
Kyoto UniversityResearch Topics
We conduct research on the mechanisms of sedimentary layer formation. Our main interest lies in the dynamics and depositional processes of sediment gravity flows. By combining field surveys, microstructural observations, tank experiments, and numerical simulations, we aim to integrate sedimentology and morphodynamics to discover new perspectives on Earth's processes.
Sediment Gravity Flow
When we explore the deep seafloor at depths of over 1,000 meters,
we often find astonishing landscapes resembling giant rivers and alluvial fans.
These features can stretch for hundreds of kilometers. Along the continental slope,
submarine canyons develop, extending into deep-sea rivers (submarine channels) that meander
endlessly.
Just like rivers on land, these submarine channels have natural levees and abandoned
channels (like oxbow lakes).
But what exactly flows through these deep-sea "rivers," surrounded entirely by seawater?
In fact, these submarine channels are believed to have been shaped by a rare phenomenon
known as
"sediment gravity flows," which occur only once every few years to hundreds of years.
A sediment gravity flow is essentially a flow of "turbid water" underwater.
For example, when an underwater landslide occurs, or when heavy rain and flooding
cause muddy water to flow into the sea, sand and mud become suspended in the water.
On land, sand dust particles quickly settle back to the ground,
but in water, buoyancy and viscosity prevent sand and mud from settling easily.
Because turbid water contains sand and mud, it becomes denser than
the surrounding seawater and starts flowing downslope under the force of
gravity—this is a sediment gravity flow.
Once it starts moving, turbulence further suspends seafloor sediments,
and collisions between sand and mud particles help maintain the turbidity for longer
periods.
As a result, sediment gravity flows can sometimes travel for hundreds of kilometers
underwater.
These flows can reach speeds of 18 meters per second (65 kilometers per hour),
and there are records of them cutting submarine cables while continuing to flow for over 13
hours.
Through this process, deep-sea landscapes that resemble rivers on land are formed.
The deep ocean remains a largely unexplored world,
where dynamic natural phenomena beyond our imagination unfold.
If you're fascinated by the mysteries of the sea and the dynamic movements of our planet,
this is a field worth exploring!
Tsunami
Tsunamis are generated by uplift movements during earthquakes,
as well as mass movements associated with volcanic eruptions and landslides.
Since tsunamis travel faster in deeper water, a wave of about one meter
near a trench can grow to several tens of meters near the coast as trailing waves catch up.
Recently, significant damage was caused by tsunami waves following the 2011 Tōhoku
earthquake
and the 2024 Noto Peninsula earthquake in Japan.
Japan is surrounded by tectonic faults capable of generating earthquakes and tsunamis,
including subduction zones such as the Kuril Trench, Japan Trench, Sagami Trough, Nankai
Trough, and Ryukyu Trench,
as well as numerous active faults near the coast of the Japan Sea.
It is known that earthquakes triggering tsunamis occur repeatedly, making it crucial to
study past seismic activity to prepare for future earthquake and tsunami disasters.
Methods for investigating past earthquakes and tsunamis include observational records,
historical documents, and geological evidence.
However, since the recurrence intervals of giant earthquakes from hundreds to thousands of
years,
geological traces must be examined for periods beyond the reach of historical records.
A key piece of evidence for past tsunamis is tsunami deposits.
These deposits consist of gravel, sand, and mud transported by tsunamis, often sourced from
beach erosion.
Finer tsunami deposits can be carried up to the inundation limit,
allowing researchers to determine past tsunami flooding extents by analyzing and correlating
sediment layers.
However, due to the diverse coastal geomorphology and sedimentary environments,
many aspects of how different tsunamis generate specific sediment deposits remain
unresolved.
We investigate deposits from modern tsunamis, such as the 2004 Sumatra, 2011 Tōhoku, and
2024 Noto events,
to examine how sediment characteristics reflect tsunami hydrodynamics.
In particular, we are developing a method that combines numerical simulations and
deep learning to estimate tsunami flow velocity, wave height, and inundation distance from
the thickness and grain size distribution of tsunami deposits.
By reconstructing the largest past tsunamis and earthquakes from sediment records,
this research may contribute to improving future natural disaster predictions.
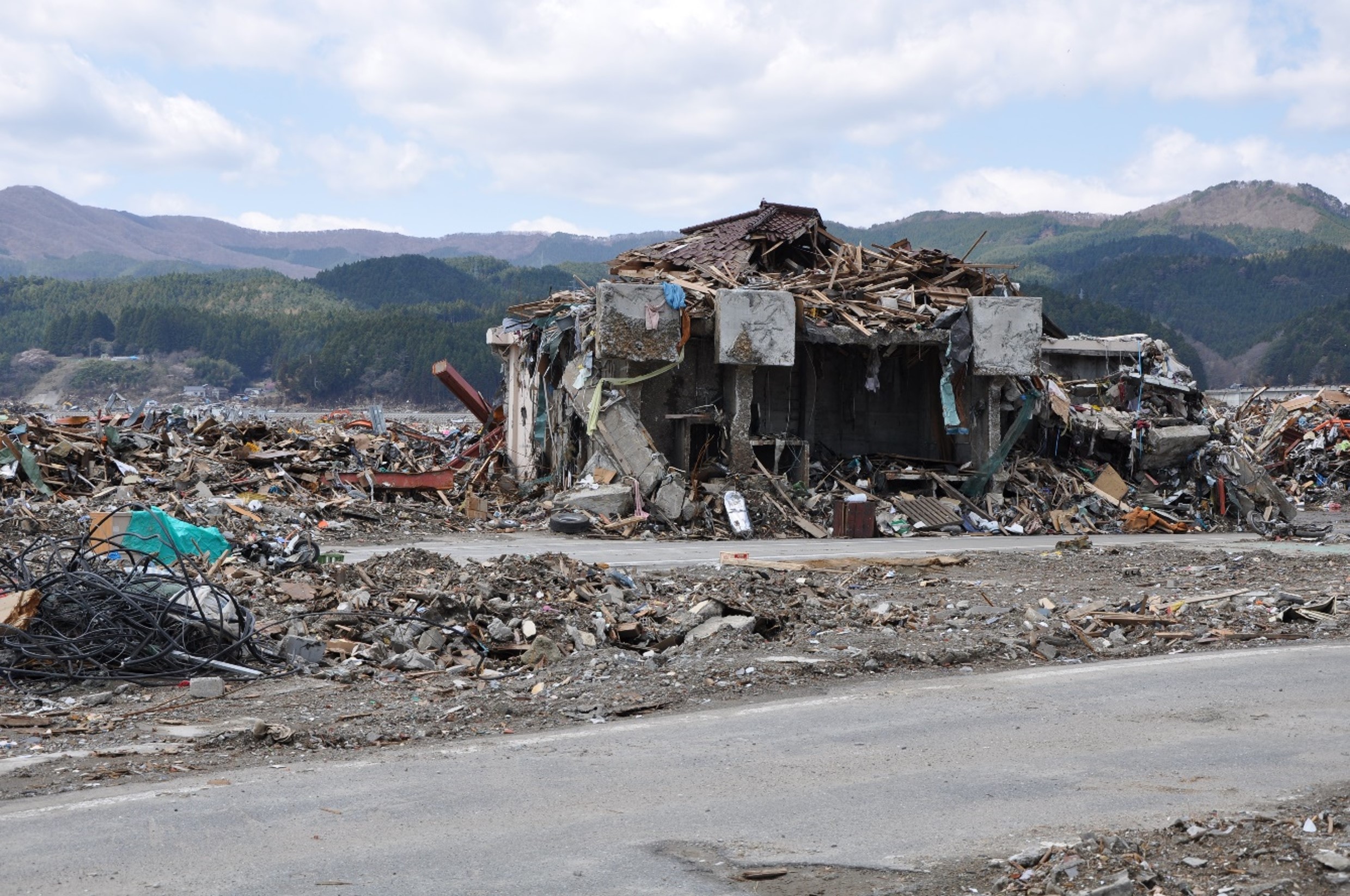
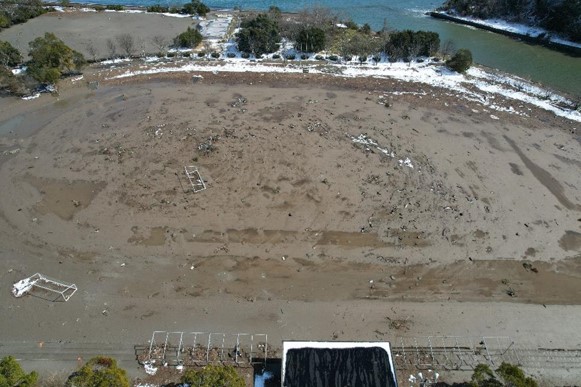
Drone picture
Bedrock River
The Earth's surface is constantly changing—mountains rise, earthquakes occur, and river
courses shift.
Behind these phenomena lies a process known as crustal uplift, a slow upward movement
of the Earth's crust.
Understanding uplift is key to deciphering earthquakes, volcanic activity, and even past
climate changes.
Japan experiences significant crustal movement due to its active seismic and volcanic
activity.
Studying how the Japanese archipelago has moved over time is crucial for understanding its
geological history.
Various methods have been used to investigate past crustal movements,
but existing techniques have struggled to provide long-term, large-scale uplift
histories.
Recently, researchers have focused on fluvial geomorphology—the study of river
landforms.
Rivers flow from mountains to the sea, and their courses and bedforms are shaped by crustal
uplift and erosion.
By modeling how rivers erode the landscape based on physical processes, we can reconstruct
past crustal movements.
One widely used forward model for reconstructing uplift history is the stream power
model.
This model assumes that river discharge and bed slope determine the rate of bedrock
incision.
By refining this approach with statistical techniques such as the Markov Chain Monte
Carlo (MCMC) method,
researchers have been able to estimate crustal uplift history with greater accuracy.
Our research applies bedrock river erosion models, such as the stream power model,
to Japanese rivers for the first time.
So far, we have estimated uplift histories in regions such as the Kii Peninsula, Shikoku,
and Tōhoku.
Moving forward, we aim to apply more complex models to better understand
the crustal dynamics of the Japanese archipelago.
Earth sciences explore how our planet moves and evolves.
By studying familiar natural phenomena—like river flows and mountain heights—
we can uncover the fundamental mechanisms that shape the Earth.
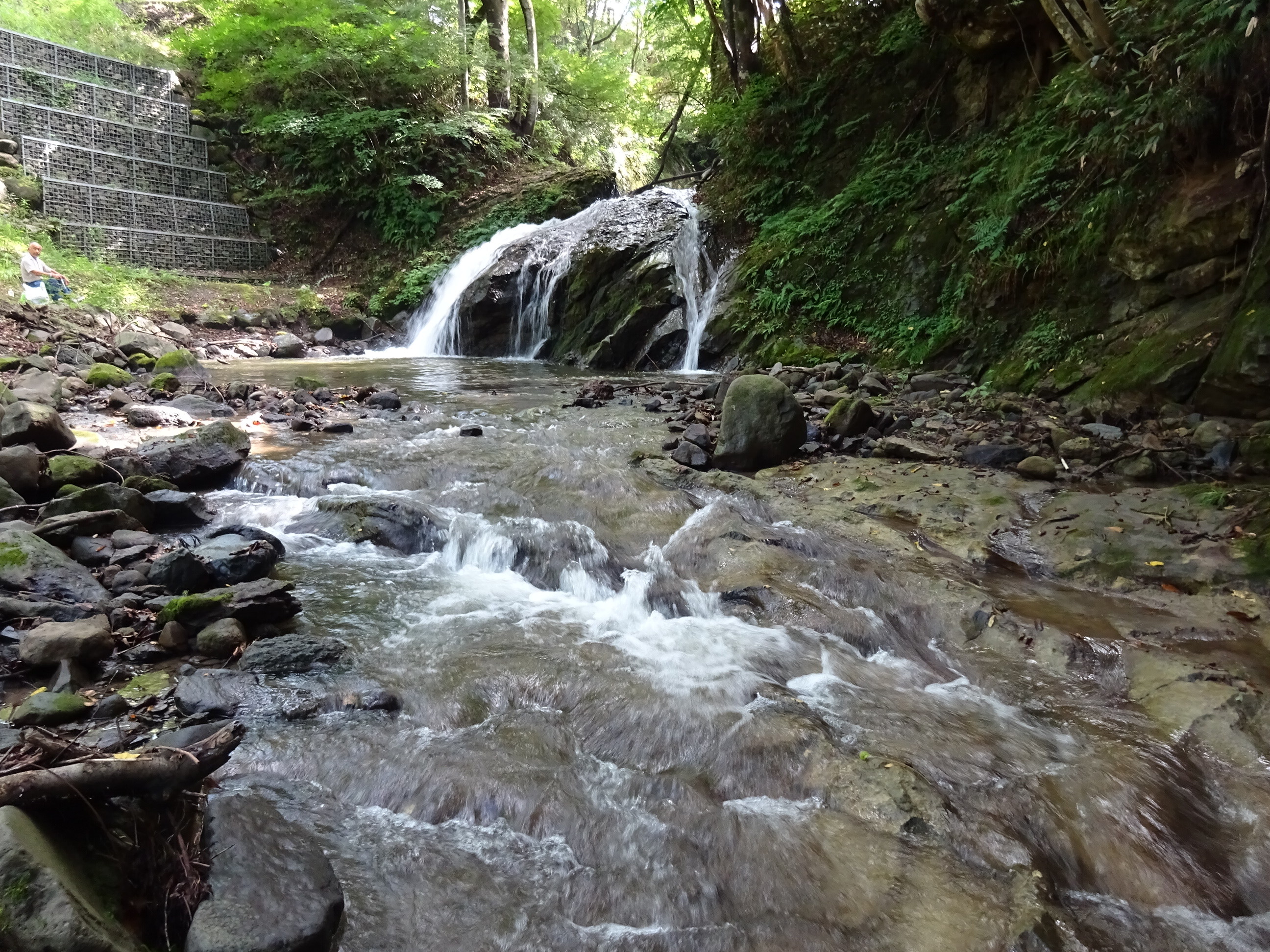
Bedform
On riverbeds and seafloors, landforms known as bedforms are
created by the interaction between water flow and sediment transport.
Ripples and dunes are classic examples,
and their shape and size vary depending on flow velocity and sediment grain size.
Bedforms can range from just a few millimeters to several hundred meters in scale.
For example, ripples and dunes that form on riverbeds develop in relatively slow-flowing
water.
Meanwhile, in the deep sea, gravity-driven turbidity currents can create bedforms that
migrate in the opposite direction of the flow.
Understanding bedform types and formation conditions is crucial in geology and Earth
sciences.
By analyzing bedform traces in sedimentary layers,
scientists can infer past water flows and environmental conditions.
For instance, sediment layers tilted downstream indicate the migration of ripples and dunes,
while parallel layers suggest stable flow conditions.
Bedform research also plays a role in river management.
Since riverbed topography affects water resistance,
predicting bedform formation can aid in flood prevention and water resource management.
Recent studies have increasingly focused on unusual bedforms that were previously
overlooked.
Examples include bedforms that develop in mixed mud-and-sand environments,
as well as similar landforms observed on Mars and other planets.
These discoveries expand our understanding of geomorphic processes and
provide a more comprehensive view of landform development.
The study of bedforms unravels the natural patterns created by water and sediment,
offering insights into Earth's and other planets' histories.
If you're fascinated by Earth's mysteries and natural phenomena,
this field is well worth exploring!
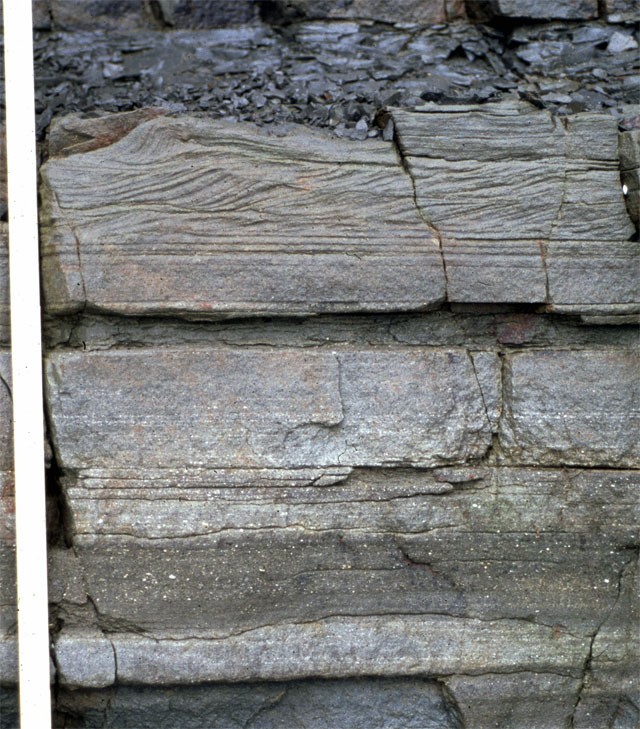
Bioturbation
Did you know that ancient creatures left their footprints on the seafloor,
preserved in rock for millions of years?
These traces, known as trace fossils, are the preserved evidence of
biological activity rather than the organisms themselves.
By studying trace fossils, we can uncover clues about past environments and
how ancient organisms lived.
One fascinating phenomenon related to trace fossils is bioturbation.
This occurs when benthic organisms burrow, feed, or move through mud and sand,
mixing the sediments in the process.
By analyzing the extent of bioturbation, scientists can infer past seafloor conditions,
such as sedimentation rates, organic matter supply, and biodiversity.
However, studying bioturbation traces in detail is a challenging task.
Identifying specific trace fossils within layers of rock and accurately measuring
their distribution and abundance requires significant time and effort.
To address this, researchers are exploring the use of image analysis technology
to automatically detect trace fossils and efficiently quantify bioturbation intensity.
This approach allows for the rapid collection of large datasets,
enabling a deeper understanding of past environmental changes and biological activity
patterns.
The study of trace fossils and bioturbation is crucial for understanding Earth's history,
the evolution of life, and the interactions between organisms and their environments.
If you're interested in Earth sciences or biology, this field offers exciting
opportunities to decode the messages left behind by ancient life.
Who knows—you might uncover a groundbreaking discovery hidden in the fossilized footprints
of the past!
Machine Learning
Have you ever heard of artificial intelligence (AI) and deep learning?
These cutting-edge technologies enable computers to learn patterns
from vast amounts of data and solve complex problems.
Surprisingly, AI is also revolutionizing sedimentology—the study of Earth's geological
history
through sediments—by helping scientists uncover past environmental changes and
detect traces of natural disasters like earthquakes and tsunamis.
We integrate deep learning into sedimentary research.
By inputting images of sediment layers and rocks into deep learning models,
we can automatically extract and classify features.
This allows for faster and more accurate sedimentary analysis than traditional manual
methods.
Some key achievements include:
- Automatic detection of sedimentary structures:
Using deep learning, we can analyze sedimentary images to identify and count trace fossils
(such as ancient footprints or burrows) and transported sand grains.
This helps us efficiently reconstruct past biological activity and depositional
environments.
- Estimation of paleo-hydraulic conditions:
By combining data on tsunami and turbidity current deposits with numerical modeling,
we can estimate past flow velocities and magnitudes.
This provides a detailed record of historical natural disasters.
- Classification of sedimentary layers and landforms:
Deep learning models trained on large datasets of stratigraphic cross-sections and
geomorphological features can quantitatively classify different sedimentary environments.
This enhances our ability to reconstruct Earth's environmental and hydrological history.
These AI-driven advances are transforming sedimentology, making complex data analysis
more efficient than ever before.
Not only does this open up new possibilities in Earth sciences, but it also contributes
to disaster prediction and mitigation efforts.
If you're interested in AI or geoscience, why not explore this cutting-edge field?
By combining deep learning with Earth science data, we are unlocking new discoveries and
insights about our planet’s past and future!
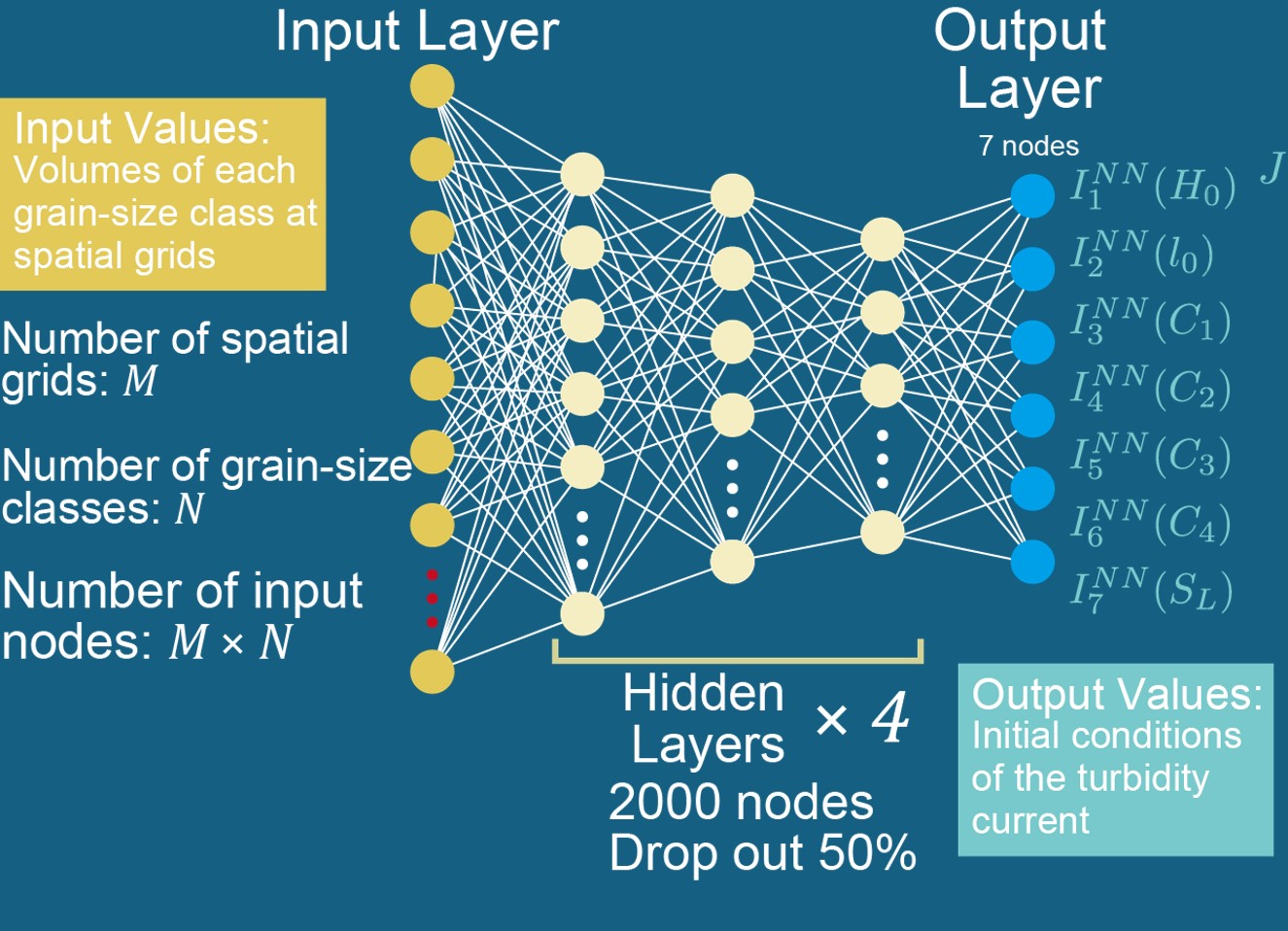
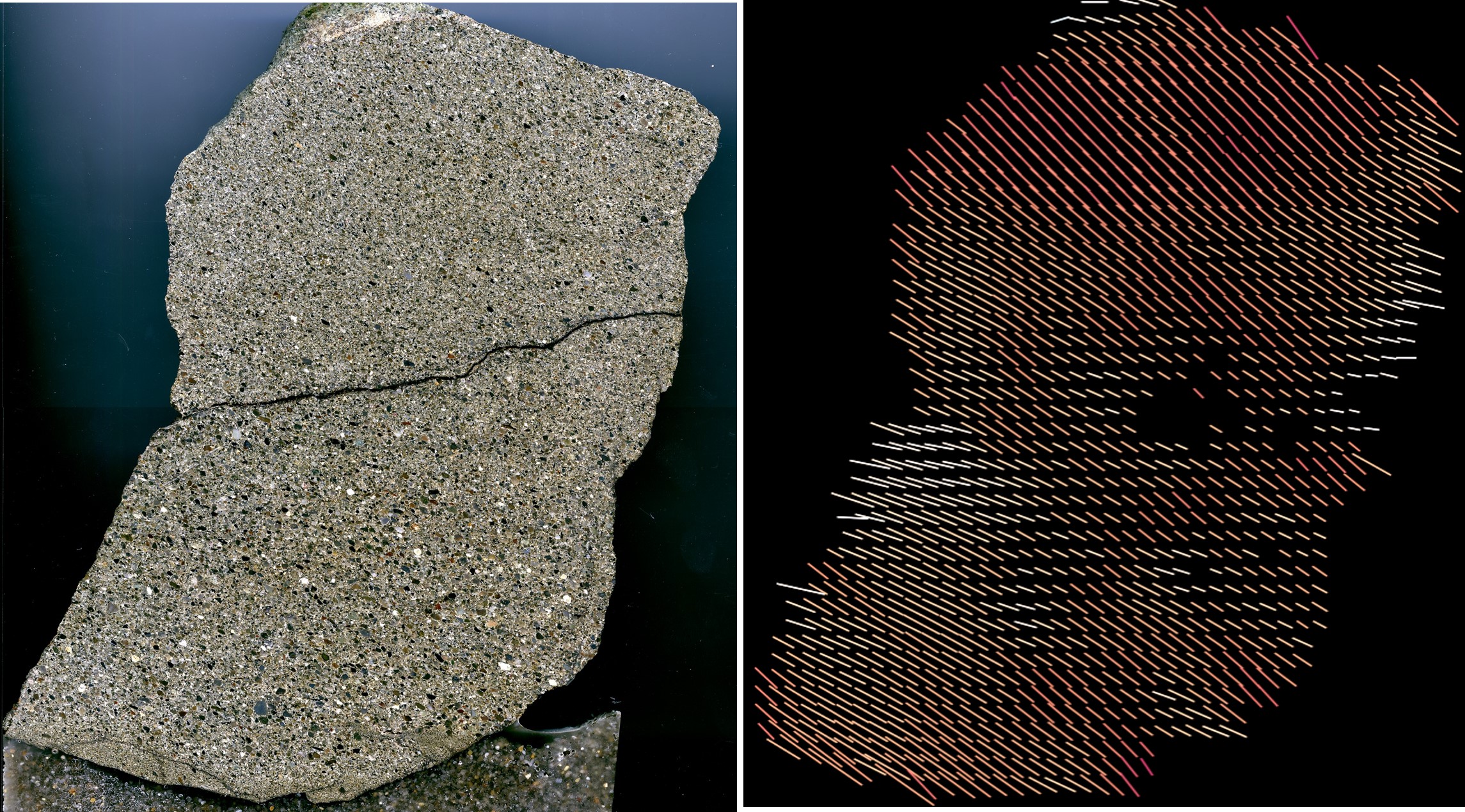